Understanding the Baby Microbiome: A Comprehensive Guide
The human microbiome, a complex community of microorganisms residing in and on our bodies, plays a critical role in health and disease. In infants, the microbiome undergoes significant changes during the first year of life, influenced by factors such as delivery mode, gestational age, and environmental exposures. This blog post delves into the intricacies of the baby microbiome, drawing insights from key studies to understand its development and implications for infant health.
The Infant Microbiome: An Overview
The infant microbiome begins to develop at birth, with initial colonization influenced by the mode of delivery. Vaginally delivered infants are exposed to their mother's vaginal and intestinal microbiota, while cesarean-delivered infants are more likely to be colonized by skin-associated microbes. This early microbial exposure is crucial as it sets the foundation for the infant's immune system and overall health .
The Role of Delivery Mode
The mode of delivery significantly impacts the initial microbial exposure and colonization patterns in newborns. Vaginal delivery results in a microbiota similar to the mother's vaginal flora, predominantly featuring Lactobacillus and Prevotella species. In contrast, cesarean section deliveries lead to a microbiota resembling the maternal skin, with a higher prevalence of Staphylococcus and Corynebacterium species .
This difference in microbial colonization can have long-term health implications. Studies suggest that cesarean-delivered infants may have an increased risk of developing conditions such as asthma, allergies, and obesity, potentially due to the altered microbial exposure at birth .
Development Across Body Sites
The infant microbiome is not uniform across the body; it varies significantly between different sites such as the skin, gut, and oral cavity. Each site provides a unique environment that shapes the microbial community structure and function.
Skin Microbiome
The skin microbiome is one of the first to be established and undergoes rapid changes during infancy. The diversity of the skin microbiome early in life is influenced by factors such as age, environment, and maternal microbiota. The skin of newborns is initially colonized by bacteria from the Firmicutes and Actinobacteria phyla, with Staphylococcus being a dominant genus .
Recent studies have further explored the development of the cutaneous microbiome in preterm infants, revealing that gestational age and antibiotic exposure significantly affect microbial diversity and richness. Preterm infants, with their underdeveloped skin barrier and immune system, often exhibit lower microbial diversity, which may predispose them to infections and other health complications .
Gut Microbiome
The gut microbiome plays a pivotal role in digestion, nutrient absorption, and immune function. In infants, the gut microbiome is highly dynamic, influenced by factors such as breastfeeding, formula feeding, and antibiotic use. Breastfed infants typically have a gut microbiome rich in Bifidobacterium species, which are associated with beneficial health outcomes such as enhanced immune function and reduced risk of gastrointestinal infections .
Microbiome Maturation and Health Implications
The maturation of the infant microbiome is a critical process that can influence long-term health. A well-balanced microbiome supports the development of a robust immune system, while dysbiosis, or microbial imbalance, has been linked to various health issues, including allergies, autoimmune diseases, and metabolic disorders.
Immune System Development
The microbiome plays a crucial role in educating the infant's immune system. Early microbial exposure helps the immune system distinguish between harmful and harmless stimuli, reducing the risk of allergic and autoimmune conditions. Recent research has emphasized the importance of microbial diversity in promoting immune tolerance and preventing overactive immune responses .
Potential Interventions
Understanding the factors that influence the infant microbiome opens avenues for potential interventions to promote healthy microbial development. Probiotics, prebiotics, and synbiotics are being explored as strategies to enhance microbial diversity and support immune function in infants, particularly those born via cesarean section or exposed to antibiotics.
Recent Advances in Baby Microbiome Research
Recent studies have provided new insights into the development and importance of the infant microbiome:
A 2023 study highlighted the potential of the microbiome as a next-generation indicator for pregnancy complications and infant health outcomes. This research suggests that analyzing the maternal and infant microbiome could lead to improved prediction and diagnosis of various pregnancy-related issues .
Researchers have found that the introduction of solid foods, particularly those rich in fibers and proteins, significantly increases the diversity of the gut microbiota in nine-month-old infants. This underscores the importance of diet in shaping the infant microbiome .
In a groundbreaking development, scientists at the University of Chicago have created an AI tool that models the infant microbiome. This virtual model could help predict how various factors, including diet and environmental exposures, might affect the development of an infant's microbiome, potentially leading to personalized interventions for optimal health .
Conclusion
The baby microbiome is a complex and dynamic ecosystem that plays a vital role in shaping infant health. From the mode of delivery to environmental exposures and dietary factors, various elements influence its development. By understanding these influences, we can better support healthy microbiome maturation and potentially mitigate the risk of health issues later in life.
As research continues to unravel the intricacies of the infant microbiome, it becomes increasingly clear that fostering a healthy microbiome from birth is crucial for long-term health and well-being. Parents and healthcare providers can play a pivotal role in supporting this process through informed choices and interventions.
References:
Dominguez-Bello MG, et al. "Delivery mode shapes the acquisition and structure of the initial microbiota across multiple body habitats in newborns." Proc Natl Acad Sci USA. 2010;107(26):11971-11975. DOI: 10.1073/pnas.1002601107.
Chu DM, et al. "The early infant gut microbiome varies in association with a cesarean section." Nat Med. 2017 Mar;23(3):314-326. DOI: 10.1038/nm.4274.
Sevelsted A, et al. "Cesarean Section and Chronic Immune Disorders." Pediatrics. 2015 Apr;135(4)
. DOI: 10.1542/peds.2014-0596.
Capone KA, et al. "Diversity of the human skin microbiome early in life." J Invest Dermatol. 2011 Oct;131(10):2026-32. DOI: 10.1038/jid.2011.168.
Pammi M, et al. "The effects of gestational age, mode of delivery, and antibiotic exposure on the microbiome of preterm infants." PLoS One. 2017;12(4)
. DOI: 10.1371/journal.pone.0176669.
Stewart CJ, et al. "Temporal development of the gut microbiome in early childhood from the TEDDY study." Nature. 2018 Oct;562(7728):583-588. DOI: 10.1038/s41586-018-0617-x.
Wopereis H, et al. "The first thousand days - intestinal microbiology of early life: establishing a symbiosis." Pediatr Allergy Immunol. 2014 May;25(5):428-438. DOI: 10.1111/pai.12232.
Pinto E, et al. "The Role of the Microbiome in Pregnancy Complications and Adverse Infant Health Outcomes: Insights from Recent Research." Nutrients. 2023;15(6):1473. DOI: 10.3390/nu15061473.
Yin et al. "Introduction of Solid Foods and Gut Microbiota in Infants: Influence on Microbial Diversity and Health Outcomes." Nutrients. 2024;16(3):400. DOI: 10.3390/nu16030400.
University of Chicago. "New AI tool models the infant microbiome, offering personalized insights." Medical Xpress. 2024 Apr 10. URL: https://medicalxpress.com/news/2024-04-ai-tool-virtual-infant-microbiome.html.
The Microbiome-Inflammation Connection: A Basic Overview
The human gut microbiome, consisting of trillions of microorganisms living in our digestive tract, plays a crucial role in our overall health. One of its most significant impacts is on our immune system and inflammatory responses. The relationship between the gut microbiome and inflammation is complex and bidirectional, with each influencing the other in various ways.
Understanding Inflammation
Inflammation is the body's natural response to injury or infection. It's a protective mechanism designed to eliminate harmful stimuli and initiate the healing process. However, when inflammation becomes chronic, it can contribute to various diseases, including inflammatory bowel disease (IBD), diabetes, and cardiovascular disease.
The Gut Microbiome's Role in Regulating Inflammation
The gut microbiome helps regulate inflammation through several mechanisms:
1. Barrier Function: A healthy gut microbiome maintains the integrity of the intestinal barrier. This barrier prevents harmful substances from entering the bloodstream and triggering systemic inflammation.
2. Immune System Education: The gut microbiome plays a crucial role in educating and modulating the immune system. It helps the immune system distinguish between harmful pathogens and beneficial microbes, preventing unnecessary inflammatory responses.
3. Production of Anti-inflammatory Compounds: Certain gut bacteria produce metabolites with anti-inflammatory properties. For example, short-chain fatty acids (SCFAs) like butyrate have been shown to have potent anti-inflammatory effects.
4. Regulation of Pro-inflammatory Compounds: The microbiome can also influence the production and circulation of pro-inflammatory compounds. For instance, some gut bacteria can metabolize certain dietary components into pro-inflammatory substances.
Dysbiosis and Inflammation
Dysbiosis, an imbalance in the gut microbial community, has been linked to increased inflammation and various inflammatory diseases. When the balance of beneficial and potentially harmful bacteria is disrupted, it can lead to:
1. Increased Intestinal Permeability: Also known as "leaky gut," this condition allows more substances to pass through the intestinal wall, potentially triggering immune responses and inflammation.
2. Altered Immune Responses: Dysbiosis can lead to inappropriate immune responses, including increased production of pro-inflammatory cytokines.
3. Changes in Metabolite Production: An imbalanced microbiome may produce fewer anti-inflammatory compounds and more pro-inflammatory ones.
Specific Inflammatory Conditions Linked to the Microbiome
Several inflammatory conditions have been associated with alterations in the gut microbiome:
1. Inflammatory Bowel Disease (IBD): Patients with IBD, including Crohn's disease and ulcerative colitis, often show significant alterations in their gut microbiome composition.
2. Obesity and Metabolic Syndrome: These conditions are characterized by low-grade chronic inflammation, which has been linked to changes in the gut microbiome.
3. Rheumatoid Arthritis: Studies have found differences in the gut microbiome of individuals with rheumatoid arthritis compared to healthy controls.
4. Cardiovascular Disease: The gut microbiome's role in producing certain metabolites has been linked to inflammation associated with cardiovascular disease.
Dietary Influences on the Microbiome and Inflammation
Diet plays a crucial role in shaping the gut microbiome and, consequently, inflammatory responses:
1. Fiber: A diet high in fiber promotes the growth of beneficial bacteria that produce anti-inflammatory compounds like SCFAs.
2. Processed Foods: Diets high in processed foods and low in fiber have been associated with dysbiosis and increased inflammation.
3. Omega-3 Fatty Acids: These have anti-inflammatory properties and can positively influence the gut microbiome.
4. Polyphenols: Found in fruits, vegetables, and tea, polyphenols can promote the growth of beneficial bacteria and have anti-inflammatory effects.
Therapeutic Approaches Targeting the Microbiome-Inflammation Axis
Understanding the microbiome-inflammation connection has led to several therapeutic approaches:
1. Probiotics: These are live beneficial bacteria that, when administered in adequate amounts, can confer health benefits, including potential anti-inflammatory effects.
2. Prebiotics: These are dietary components that feed beneficial gut bacteria, potentially promoting an anti-inflammatory gut environment.
3. Fecal Microbiota Transplantation (FMT): This involves transferring fecal matter from a healthy donor to a recipient, with the aim of restoring a healthy microbial balance.
4. Diet Modification: Adopting diets rich in fiber, omega-3 fatty acids, and polyphenols can positively influence the gut microbiome and reduce inflammation.
Conclusion
The relationship between the gut microbiome and inflammation is intricate and multifaceted. A healthy, diverse microbiome plays a crucial role in maintaining immune balance and preventing chronic inflammation. Conversely, dysbiosis can contribute to inflammatory processes and various associated diseases. As our understanding of this relationship deepens, it opens up new avenues for preventing and treating inflammatory conditions through microbiome-targeted approaches. However, much remains to be learned about the specific mechanisms involved and how to effectively manipulate the microbiome for optimal health outcomes.
Sources
[1] Long-term dietary patterns are associated with pro-inflammatory and ... https://gut.bmj.com/content/70/7/1287
[2] The Gut Microbiota and Inflammation: An Overview - PMC - NCBI https://www.ncbi.nlm.nih.gov/pmc/articles/PMC7589951/
[3] The Gut Microbiome and Inflammatory Bowel Diseases - PMC - NCBI https://www.ncbi.nlm.nih.gov/pmc/articles/PMC10012812/
[4] Role of gut microbiota in infectious and inflammatory diseases https://www.frontiersin.org/journals/microbiology/articles/10.3389/fmicb.2023.1098386/full
[5] Immunological mechanisms of inflammatory diseases caused by gut ... https://www.sciencedirect.com/science/article/pii/S0753332223007758
Phylum Dive: Firmicutes
Firmicutes is a one of the major phyla in the human gut, having the highest abundance of gut bacteria. The phylum consists of many beneficial bacteria but also some problematic bacteria at higher levels compared to the healthy cohort. For example, high levels of certain species of Firmicutes have been seen in patients with obesity, type II diabetes, and Irritable Bowel Syndrome. Other bacteria belonging to Firmicutes are major producers of short chain fatty acids that have many beneficial health implications: protection against Inflammatory Bowel Disease, diabetes, obesity, and cancer.
Firmicutes Keystone Species
Keystone species are bacteria that play crucial roles in the human gut, even though they aren’t necessarily seen at high abundances.
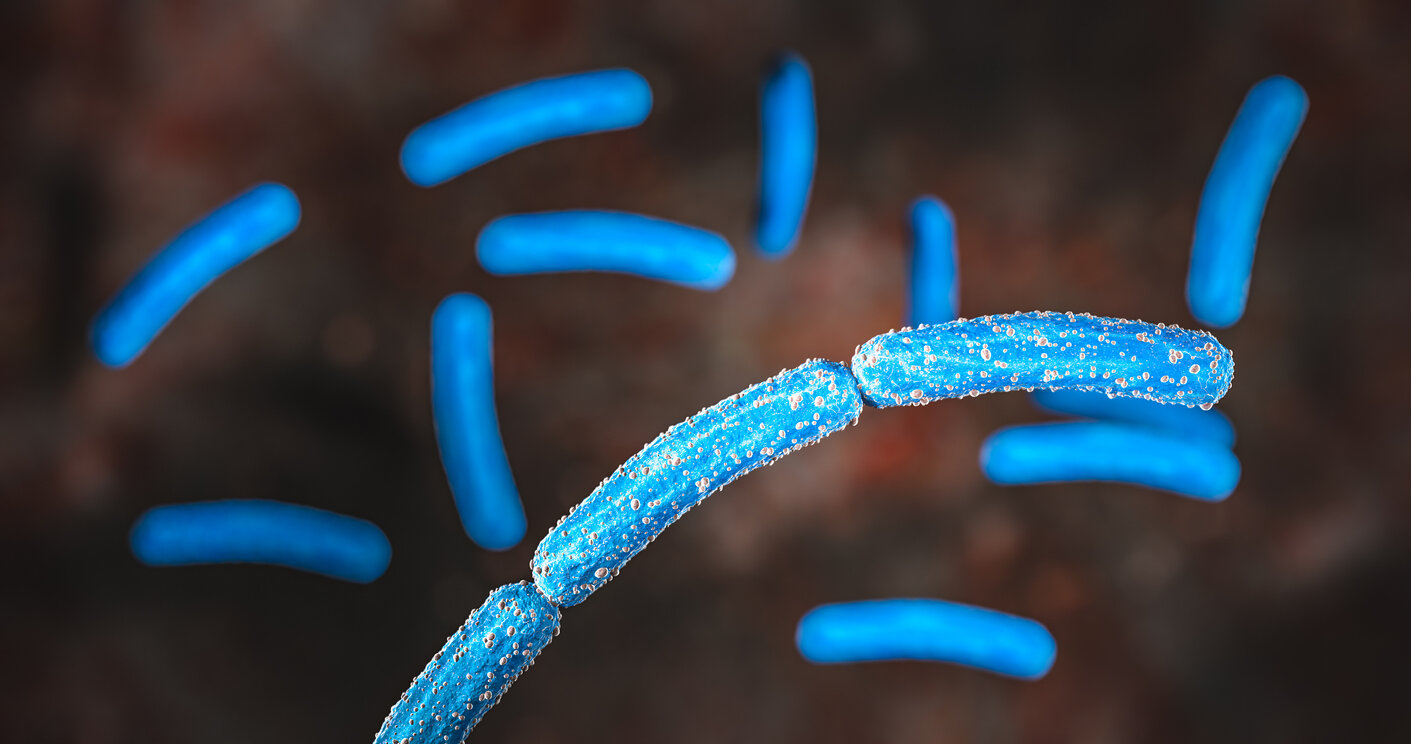
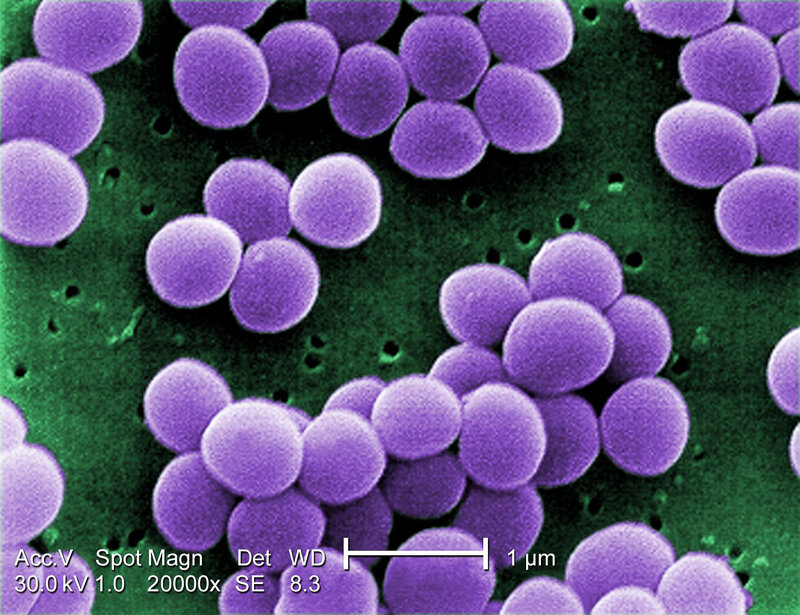
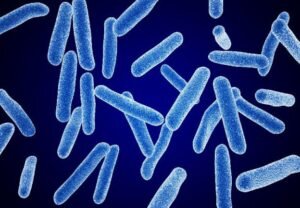
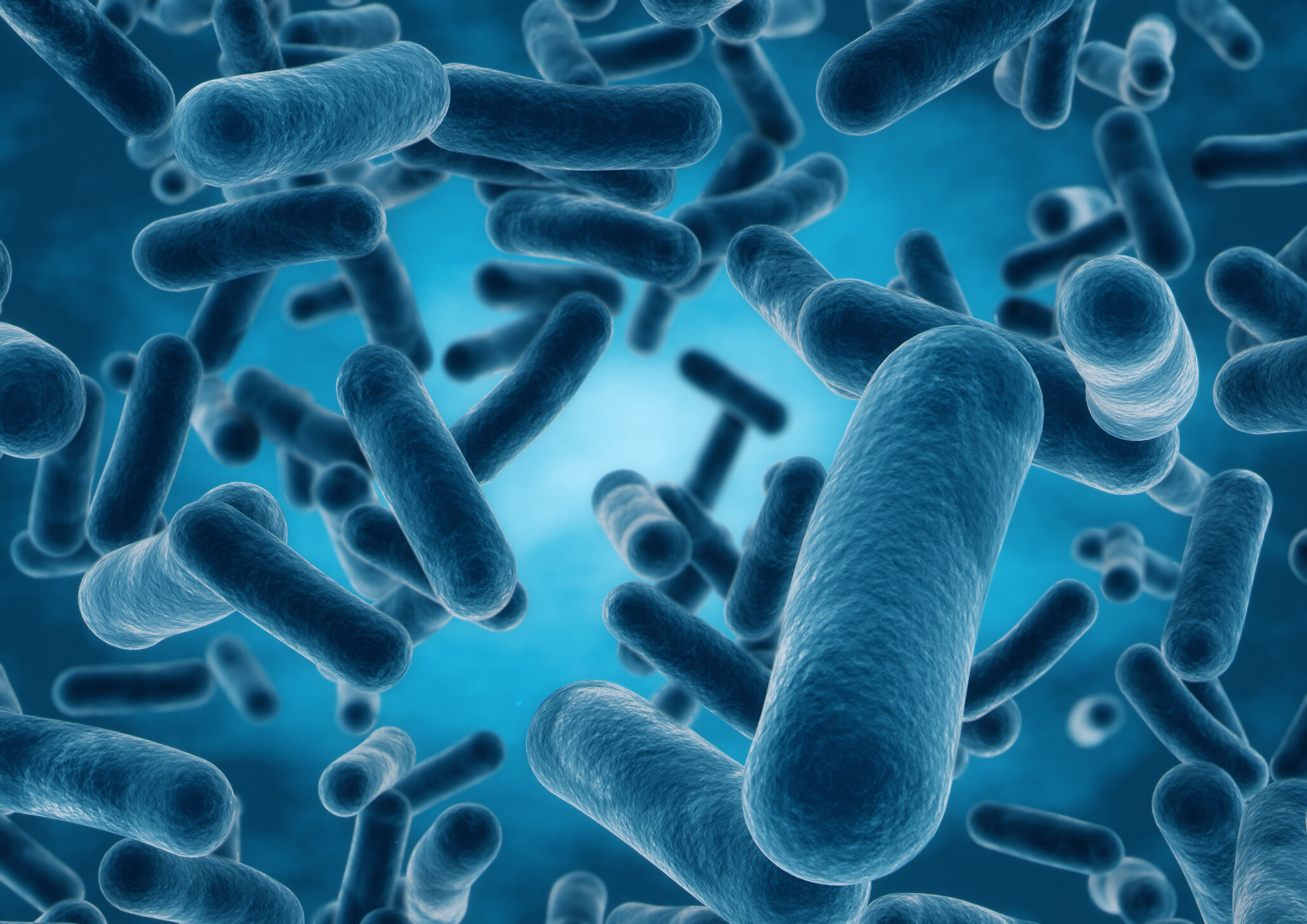
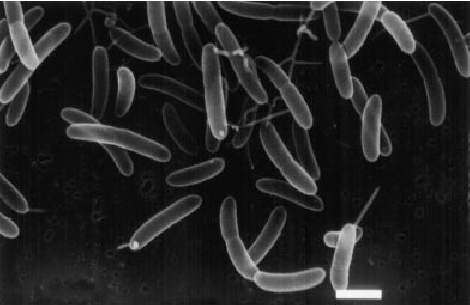
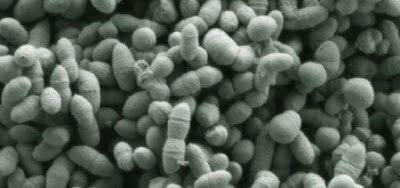
As seen above:
Bacillus spp. have been known to have antimicrobial properties making them beneficial to human health.
Eubacterium rectale is a butyrate producer. It also plays an important role in the fermentation of amino acids and uptake of sugars. Increasing your intake of fruits, vegetables and fibers can increase your Eubacterium rectale levels.
Faecalibacterium prausnitzii is the most abundant microbe in the human gut, and lower levels of Faecalibacterium prausnitzii compared to the healthy cohort have been noted in individuals with Crohn's Disease. Because Faecalibacterium prausnitzii can use many types of sugars and amino acids, it is able to produce short chain fatty acids such as butyrate, formate, and lactate. Faecalibacterium prausnitzii is important in maintaining homeostasis in the gut and works as an anti-inflammatory agent in mice.
Lactobacillus spp. are some of the biggest lactic acid producers; lactic acid aids in anti-microbial functions in the gut. Certain species also regulate cell death and assist the immune system. For example, Lactobacillus reuteri is used in probiotics and is helpful in killing harmful pathogens . However, compared to healthy cohorts, higher levels of Lactobacillus spp. have been seen in individuals with Autism Spectrum Disorder, but patients with Crohn's Disease show a lower abundance of beneficial Lactobacillus spp.
Roseburia spp. produce butyrate, propionate, and acetate through the fermentation of insoluble carbohydrates. They are overall a beneficial genus, with reduced levels in individuals with colorectal cancer and type II diabetes. Exercising and eating fruits, vegetables, and fibers can increase levels of Roseburia spp.
Ruminococcus spp. is a favorable genus with high abundances seen in healthy individuals. However, increased levels compared to healthy individuals have been noted in people with Irritable Bowel Syndrome. Lower abundances of Ruminococcus bromii have been seen in individuals who keep an animal-based diet; eating foods rich in fruits, vegetables, and fibers can increase levels of Ruminococcus bromii.
Works Cited:
Hasan, Nihal, and Hongyi Yang. “Factors Affecting the Composition of the Gut Microbiota, and Its Modulation.” PeerJ 7 (August 16, 2019): e7502. https://doi.org/10.7717/peerj.7502.
Jandhyala, Sai Manasa, Rupjyoti Talukdar, Chivkula Subramanyam, Harish Vuyyuru, Mitnala Sasikala, and D Nageshwar Reddy. “Role of the Normal Gut Microbiota.” World Journal of Gastroenterology : WJG 21, no. 29 (August 7, 2015): 8787–8803. https://doi.org/10.3748/wjg.v21.i29.8787.
Kagele, Dominique and Ph.D. “The ‘Skinny’ on Gut Microbes and Your Health.” The Jackson Laboratory. Accessed July 1, 2021. https://www.jax.org/news-and-insights/jax-blog/2015/may/the-skinny-on-gut-microbes-and-your-health.
Rajilić-Stojanović, Mirjana, and Willem M de Vos. “The First 1000 Cultured Species of the Human Gastrointestinal Microbiota.” Fems Microbiology Reviews 38, no. 5 (September 2014): 996–1047. https://doi.org/10.1111/1574-6976.12075.
Rinninella, Emanuele, Pauline Raoul, Marco Cintoni, Francesco Franceschi, Giacinto Abele Donato Miggiano, Antonio Gasbarrini, and Maria Cristina Mele. “What Is the Healthy Gut Microbiota Composition? A Changing Ecosystem across Age, Environment, Diet, and Diseases.” Microorganisms 7, no. 1 (January 10, 2019): 14. https://doi.org/10.3390/microorganisms7010014.
Welcome to the NirvanaBiome Blog
Welcome all microbe and microbiome lovers! We’ll try our best to keep you updated on recent developments in the field and science that’s related to microbes and the microbiome. Additionally, we would be really glad to make you smile through showing how fun, interesting, and accessible science can be in our blog. To launch our posts on microbes and the microbiome, we believe that theories on the emergence of life and our attempts to classify living organisms constitute great starting points. Therefore, our next 4 posts will give you some background on how the attempts to classify life initiated and how it led us to develop methods to characterize and classify microbes. We will then try to present different microbes and their relevance to humans and Earth. So, hold your seats, fasten your seatbelts and prepare to dive into the deepest roots of biology to start our journey into microbial life.
Theories on Emergence of Microscopic Life
It all begins with an idea.
The vast diversity of life on earth has fascinated humanity since antiquity and has driven us to develop numerous theories on the mechanisms generating diversity. Aristotle (384-322 BC) was the first person known to have a hot theory on how life diversified on earth: through spontaneous generation. Nonetheless, the invention of the microscope (1682) by Antonie von Leeuwenhoek delivered a blow to the observation of the spontaneous flourishing of life, including maggots, flies, vermin, fungi etc., from non-living things, such as rotten food, setting the framework for “Aristotelian Abiogenesis''. In 1859, the fade-in of microscopic living made Louis Pasteur test if the observation of “spontaneous generation” is due to microbes propagating in air. To test that, he boiled beef broth in an instrument of his invention that diffused air in and out but did not allow any microbial particles through. Pasteur’s boiled broth did not contain life, and confirmed the idea that diverse life on earth did not spontaneously arise from non-living things and implied that organisms arose from other organisms. Nonetheless, no one had a theory on where the microscopic life filtered out by Pasteur came from by the end of Pasteur’s experiment, not even Pasteur. By the mid 19th century, there was a gap in humanity’s understanding of how different microscopic living organisms emerged, yet bright people were already exploring ideas on the emergence of different macroscopic life either due to curiosity on human history and where humans came from or by sheer fascination by nature.
Figures 1-4 in the image show rabbit sperm and Fig. 5-8 show dog sperm observed by Leeuwenhoek (Image thanks to the permission of Wellcome Trust)
Tree of Life & Verge of Taxonomy
It all begins with an idea.
1859 was a golden year for biogenesis. Apart from Pasteur undermining “Spontaneous Generation”, Charles Darwin also published his groundbreaking book “On the Origin of Species”. The piece was successful at conceptualizing biogenesis into “Theory of Evolution”. According to evolutionary theory, the current diversity of life was generated by the living ancestors of the past. In the book, Darwin suggested that small variation in a species’ individuals’ distinguishing traits may be selected to diverge under different conditions. The divergence of traits may lead to the generation of new species or new groups of species (genus). Darwin illustrated the mode of life’s divergence as a branching tree of life (ToL) in his book.
This illustration of the branching tree of life which shows how life diversified, was the only illustration in The Origin of Species, Darwin, 1859
A classic example of natural selection’s capacity to shape different morphologies that also quite helped Darwin to establish his evolutionary theory came from Finches of Galapagos Islands during Darwin’s trip there. The morphological diversity of finches caused Darwin to not initially classify all finches that he collected during his trip as finches. Nevertheless, understanding that morphological differences of finches’ beaks were adaptations to different food sources on different Galapagos Islands led Darwin to think that all finches arose from a common ancestor that adapted to different food sources in different environments. The success of Darwin’s theory led other people to fizz with ideas to classify living organisms according to common morphological and behavioural traits or establishing novel ToLs. Classifying organisms according to common traits or similarities was an idea that was established by Carl Linnaeus in 1735, well before Darwin. Although the clades; animals, plants and minerals, where Linnaeus classified living are currently invalid, we still use the taxonomic ranking scale that he has once limited to kingdom, class, order, genus and species.
Darwin’s or Galapagos Finches, from The Voyage of Beagle, Darwin, 1845
Classification Rush
It all begins with an idea.
Darwin led lots of bright people to classify life based on their observable similarities and dissimilarities. Thanks to these attempts, numerous trees of life (ToLs) with different goals, ie. understanding the emergence of the human species or exploring major transitions in evolutionary history, were generated. 1879 was a significant year for microbial taxonomy, not just because Ernst Haeckal published another ToL putting humans to the very pinnacle of evolution, but also because he positioned microscopic amoeba to the root of ToL illustrating human evolution. Interestingly, Haeckal’s suggestion still appears true today since phylogenetic trees based on molecular techniques illustrate amoeba and animals diverging from a common ancestor. Haeckal’s ToL illustrates 2 kingdoms of the living: protists and animals. Following Haeckal’s ToL exploring microscopic organisms (Protists), Mereschkowski published another ToL suggesting that complex life forms such as animal and plants arose as a result of endosymbiosis of microscopic bacterial species, which in fact is partially correct. Today we know that eukaryotes arose as a result of an endosymbiosis between a bacterium and an archeon, and plants arose owing to an endosymbiosis between a eukaryote and a cholorophyllic bacterium thanks to developments in DNA-based molecular techniques. Hence, the lack of molecular techniques in 1905 may redeem Mereschkowski.
The ToL of Haeckal showing the evolution of human, The Evolution of Man, Haeckal, 1879
The ToL of Mereschkowski showing serial endosymbiosis that gave rise to eukaryotes and plants, Über Natur und Ursprung der Chromatophoren im Pflanzenreiche, Mereschkowski, 1905, Courtesy of Bill Martin
Nonetheless, Mereschowski neither knew what an archeon is nor that Archeae have stark similarities and dissimilarities to Bacteria. In 1925, Chatton realized that microscopic species had vast variation in their morphology and physiology, which suggested that classifying all microbes in the same domain is incorrect. Consequently, Chatton became the first to categorize all life into 2 domains, Eukaryota and Prokaryota, based on their nuclear morphology. After this division into two domains, inconsistencies due to morphology and physiology-based taxonomic attempts fueled later debates on how to branch these domains into kingdoms.
Molecular Revolution & Microbial Controversies
It all begins with an idea.
For 5 decades, Prokaryotes’ subdivision into Bacterial and Archaeal kingdoms remained unknown. Carl Woese became the first to resolve the taxonomic mystery thanks to developing 16s rRNA method classifying organisms based on sequence variation of the highly conserved and essential 16s rRNA gene. Significant divergence in prokaryotic 16s rRNA genes led Woese to divide the prokaryotic domain into archaeal and bacterial kingdoms which are also significantly divergent in physiology and ecology. Moreover, 16s rRNA method emerged to successfully assign taxa to eukaryotic organisms at a species resolution. The 16s method’s success in eukaryotic taxonomic identification, as well as prokaryotes’ subdivision into archaeal and bacterial kingdoms, led people to think about the branches of bacterial and archaeal domains into lower ranks of taxonomy, ie. phylum. Therefore, Woese attempted to identify the branch order of bacterial phyla using the 16s technique. Although the branch order of bacterial phyla is still debated, Woese’s attempt revealed that bacteria and archaea shared a common ancestor and archaea created a monophyletic group with eukaryotes. Consequently, Woese’s attempt to characterize bacterial phyla led him to divide 2 main domains (Prokaryote and Eukaryote, into 3 domains (Archaea, Bacteria and Eukarya).
Woese’s phlyogenetic ToL showing three domains of life, Towards a natural system of organisms: Proposal for the domains Archaea, Bacteria, and Eucarya, Woese et al. 1990
Woese’s successors tried to identify evolutionary bacterial phyla branches based on cell wall morphology, conserved coding mutations or conserved protein sequences. Yet, none was to have a verdict on the status of bacterial phyla branchings. Therefore, the scientific community welcomed a novel molecular method: whole-genome sequencing (WGS), to identify branchings in the lower ranks of bacterial taxonomy. WGS emerges as a more powerful technique as it compares and contrasts species’ whole genome sequences, instead of a single gene’s. Novel phylogenetic trees were also generated using WGS, but WGS arose new questions rather than resolving the issue on bacterial phyla branchings. WGS revealed that bacterial genes were interchangeable meaning that bacteria could transfer genes amongst themselves. Gene transfer amongst bacteria being common in nature, as well as some bacteria being unculturable,or cryptic in extreme environments further confounded how bacteria branched into lower phylum ranks of taxonomy. There is still an ongoing debate on bacterial phyla branching, as there is still also no common definition of what a bacterial species is. Understanding bacterial phyla branching order would resolve a mystery in evolutionary biology which may then focus our efforts on resolving bacterial taxonomy in finer ranks. To do that, it appears in order to understand what a bacterial phylum is and what is bacterial phyla’s ecological significance.